Peptide Folding, Misfolding, and Nonfolding
Wiley Series in Protein and Peptide Science
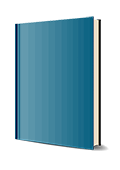
1. Edition April 2012
576 Pages, Hardcover
Wiley & Sons Ltd
Short Description
This book provides an overview on what researchers have learned about unfolded peptides and how this knowledge facilitates the understanding of (a) the folding process, (b) the binding of ligands to receptor molecules, and (c) peptide self-aggregation. In this context, different experimental, theoretical, and computational concepts and approaches are introduced. This book can become a very useful addition for graduate-level courses on protein folding for the education of undergraduate and graduate students in research groups, which are exploring peptide self-aggregation for biomedical and biotechnological purposes.
This book provides an overview on what researchers have learned about unfolded peptides and how this knowledge facilitates the understanding of (a) the folding process, (b) the binding of ligands to receptor molecules, and (c) peptide self-aggregation. In this context, different experimental, theoretical, and computational concepts and approaches are introduced. This book can become a very useful addition for graduate-level courses on protein folding for the education of undergraduate and graduate students in research groups, which are exploring peptide self-aggregation for biomedical and biotechnological purposes.
Preface xv
Contributors xix
INTRODUCTION 1
1 Why Are We Interested in the Unfolded Peptides and Proteins? 3
Vladimir N. Uversky and A. Keith Dunker
1.1 Introduction, 3
1.2 Why Study IDPs?, 4
1.3 Lesson 1: Disorderedness Is Encoded in the Amino Acid Sequence and Can Be Predicted, 5
1.4 Lesson 2: Disordered Proteins Are Highly Abundant in Nature, 7
1.5 Lesson 3: Disordered Proteins Are Globally Heterogeneous, 9
1.6 Lesson 4: Hydrodynamic Dimensions of Natively Unfolded Proteins Are Charge Dependent, 14
1.7 Lesson 5: Polymer Physics Explains Hydrodynamic Behavior of Disordered Proteins, 16
1.8 Lesson 6: Natively Unfolded Proteins Are Pliable and Very Sensitive to Their Environment, 18
1.9 Lesson 7: When Bound, Natively Unfolded Proteins Can Gain Unusual Structures, 20
1.10 Lesson 8: IDPs Can Form Disordered or Fuzzy Complexes, 25
1.11 Lesson 9: Intrinsic Disorder Is Crucial for Recognition, Regulation, and Signaling, 25
1.12 Lesson 10: Protein Posttranslational Modifi cations Occur at Disordered Regions, 28
1.13 Lesson 11: Disordered Regions Are Primary Targets for AS, 30
1.14 Lesson 12: Disordered Proteins Are Tightly Regulated in the Living Cells, 31
1.15 Lesson 13: Natively Unfolded Proteins Are Frequently Associated with Human Diseases, 33
1.16 Lesson 14: Natively Unfolded Proteins Are Attractive Drug Targets, 35
1.17 Lesson 15: Bright Future of Fuzzy Proteins, 38
Acknowledgments, 39
References, 40
I CONFORMATIONAL ANALYSIS OF UNFOLDED STATES 55
2 Exploring the Energy Landscape of Small Peptides and Proteins by Molecular Dynamics Simulations 57
Gerhard Stock, Abhinav Jain, Laura Riccardi, and Phuong H. Nguyen
2.1 Introduction: Free Energy Landscapes and How to Construct Them, 57
2.2 Dihedral Angle PCA Allows Us to Separate Internal and Global Motion, 61
2.3 Dimensionality of the Free Energy Landscape, 62
2.4 Characterization of the Free Energy Landscape: States, Barriers, and Transitions, 65
2.5 Low-Dimensional Simulation of Biomolecular Dynamics to Catch Slow and Rare Processes, 67
2.6 PCA by Parts: The Folding Pathways of Villin Headpiece, 69
2.7 The Energy Landscape of Aggregating A²-Peptides, 73
2.8 Concluding Remarks, 74
Acknowledgments, 75
References, 75
3 Local Backbone Preferences and Nearest-Neighbor Effects in the Unfolded and Native States 79
Joe DeBartolo, Abhishek Jha, Karl F. Freed, and Tobin R. Sosnick
3.1 Introduction, 79
3.2 Early Days: Random Coil--Theory and Experiment, 80
3.3 Denatured Proteins as Self-Avoiding Random Coils, 82
3.4 Modeling the Unfolded State, 82
3.5 NN Effects in Protein Structure Prediction, 86
3.6 Utilizing Folding Pathways for Structure Prediction, 87
3.7 Native State Modeling, 88
3.8 Secondary-Structure Propensities: Native Backbones in Unfolded Proteins, 92
3.9 Conclusions, 92
Acknowledgments, 93
References, 94
4 Short-Distance FRET Applied to the Polypeptide Chain 99
Maik H. Jacob and Werner M. Nau
4.1 A Short Timeline of Resonance Energy Transfer Applied to the Polypeptide Chain, 99
4.2 A Short Theory of FRET Applied to the Polypeptide Chain, 101
4.3 DBO and Dbo, 105
4.4 Short-Distance FRET Applied to the Structured Polypeptide Chain, 107
4.5 Short-Distance FRET to Monitor Chain-Structural Transitions upon Phosphorylation, 116
4.6 Short-Distance FRET Applied to the Structureless Chain, 120
4.7 The Future of Short-Distance FRET, 125
Acknowledgments, 125
Dedication, 126
References, 126
5 Solvation and Electrostatics as Determinants of Local Structural Order in Unfolded Peptides and Proteins 131
Franc Avbelj
5.1 Local Structural Order in Unfolded Peptides and Proteins, 131
5.2 ESM, 134
5.3 The ESM and Strand-Coil Transition Model, 137
5.4 The ESM and Backbone Conformational Preferences, 138
5.5 The Nearest-Neighbor Effect, 141
5.6 The ESM and Cooperative Local Structures--Fluctuating ²-Strands, 141
5.7 The ESM and ²-Sheet Preferences in Native Proteins--Significance of Unfolded State, 144
5.8 The ESM and Secondary Chemical Shifts of Polypeptides, 145
5.9 Role of Backbone Solvation in Determining Hydrogen Exchange Rates of Unfolded Polypeptides, 148
5.10 Other Theoretical Models of Unfolded Polypeptides, 148
Acknowledgments, 149
References, 149
6 Experimental and Computational Studies of Polyproline II Propensity 159
W. Austin Elam, Travis P. Schrank, and Vincent J. Hilser
6.1 Introduction, 159
6.2 Experimental Measurement of PII Propensities, 161
6.3 Computational Studies of Denatured State Conformational Propensities, 168
6.4 A Steric Model Reveals Common PII Propensity of the Peptide Backbone, 172
6.5 Correlation of PII Propensity to Amino Acid Properties, 175
6.6 Summary, 180
Acknowledgments, 180
References, 180
7 Mapping Conformational Dynamics in Unfolded Polypeptide Chains Using Short Model Peptides by NMR Spectroscopy 187
Daniel Mathieu, Karin Rybka, Jürgen Graf, and Harald Schwalbe
7.1 Introduction, 187
7.2 General Aspects of NMR Spectroscopy, 189
7.3 NMR Parameters and Their Measurement, 191
7.4 Translating NMR Parameters to Structural Information, 202
7.5 Conclusions, 213
Acknowledgments, 215
References, 215
8 Secondary Structure and Dynamics of a Family of Disordered Proteins 221
Pranesh Narayanaswami and Gary W. Daughdrill
8.1 Introduction, 221
8.2 Materials and Methods, 223
8.3 Results and Discussion, 226
Acknowledgments, 235
References, 235
II DISORDERED PEPTIDES AND MOLECULAR RECOGNITION 239
9 Binding Promiscuity of Unfolded Peptides 241
Christopher J. Oldfi eld, Bin Xue, A. Keith Dunker, and Vladimir N. Uversky
9.1 Protein-Protein Interaction Networks, 241
9.2 Role of Intrinsic Disorder in PPI Networks, 242
9.3 Transient Structural Elements in Protein-Based Recognition, 243
9.4 Chameleons and Adaptors: Binding Promiscuity of Unfolded Peptides, 256
9.5 Principles of Using the Unfolded Protein Regions for Binding, 262
9.6 Conclusions, 266
Acknowledgments, 266
References, 266
10 Intrinsic Flexibility of Nucleic Acid Chaperone Proteins from Pathogenic RNA Viruses 279
Roland Ivanyi-Nagy, Zuzanna Makowska, and Jean-Luc Darlix
10.1 Introduction, 279
10.2 Retroviruses and Retroviral Nucleocapsid Proteins, 280
10.3 Core Proteins in the Flaviviridae Family of Viruses, 288
10.4 Coronavirus Nucleocapsid Protein, 290
10.5 Hantavirus Nucleocapsid Protein, 291
Acknowledgments, 293
References, 293
III AGGREGATION OF DISORDERED PEPTIDES 307
11 Self-Assembling Alanine-Rich Peptides of Biomedical and Biotechnological Relevance 309
Thomas J. Measey and Reinhard Schweitzer-Stenner
11.1 Biomolecular Self-Assembly, 309
11.2 Misfolding and Human Disease, 310
11.3 Exploitation of Peptide Self-Assembly for Biotechnological Applications, 326
11.4 Concluding Remarks, 340
Acknowledgments, 340
References, 340
12 Structural Elements Regulating Interactions in the Early Stages of Fibrillogenesis: A Human Calcitonin Model System 351
Rosa Maria Vitale, Giuseppina Andreotti, Pietro Amodeo, and Andrea Motta
12.1 Stating the Problem, 351
12.2 Aggregation Models: The State of The Art, 354
12.3 Human Calcitonin hCT as a Model System for Self-Assembly, 356
12.4 The "Prefi brillar" State of hCT, 358
12.5 How Many Molecules for the Critical Nucleus?, 361
12.6 Modeling Prefi brillar Aggregates, 366
12.7 hCT Helical Oligomers, 366
12.8 The Role of Aromatic Residues in the Early Stages of Amyloid Formation, 372
12.9 The Folding of hCT before Aggregation, 373
12.10 Model Explains the Differences in Aggregation Properties between hCT and sCT, 374
12.11 hCT Fibril Maturation, 375
12.12 ±-Helix -->²-Sheet Conformational Transition and hCT Fibrillation, 377
12.13 Concluding Remarks, 378
Acknowledgments, 378
References, 379
13 Solution NMR Studies of A² Monomers and Oligomers 389
Chunyu Wang
13.1 Introduction, 389
13.2 Overexpression and Purifi cation of Recombinant A², 390
13.3 A² Monomers, 393
13.4 A² Oligomers and Monomer-Oligomer Interaction, 403
13.5 Conclusion, 406
References, 406
14 Thermodynamic and Kinetic Models for Aggregation of Intrinsically Disordered Proteins 413
Scott L. Crick and Rohit V. Pappu
14.1 Introduction, 413
14.2 Thermodynamics of Protein Aggregation--the Phase Diagram Approach, 415
14.3 Thermodynamics of IDP Aggregation (Phase Separation)--MPM Description, 420
14.4 Kinetics of Homogeneous Nucleation and Elongation Using MPMs, 425
14.5 Concepts from Colloidal Science, 427
14.6 Conclusions, 433
Acknowledgments, 433
References, 434
15 Modifiers of Protein Aggregation--From Nonspecific to Specific Interactions 441
Michal Levy-Sakin, Roni Scherzer-Attali, and Ehud Gazit
15.1 Introduction, 441
15.2 Nonspecific Modifi ers, 442
15.3 Specific Modifiers, 454
Acknowledgments, 465
References, 466
16 Computational Studies of Folding and Assembly of Amyloidogenic Proteins 479
J. Srinivasa Rao, Brigita Urbanc, and Luis Cruz
16.1 Introduction, 479
16.2 Amyloids, 480
16.3 Computer Simulations, 485
16.4 Summary, 514
References, 515
INDEX 529